Users
Social media
- More details here...
- Address
Parc Científic de la Universitat de València C/
Catedrático Agustín Escardino, 9
46980 Paterna (Valencia) Spain - Email:
iu.i2sysbio@uv.es - Phone:
(+34) 963544810
- Address
Links
Viruses have a secret, altruistic social life
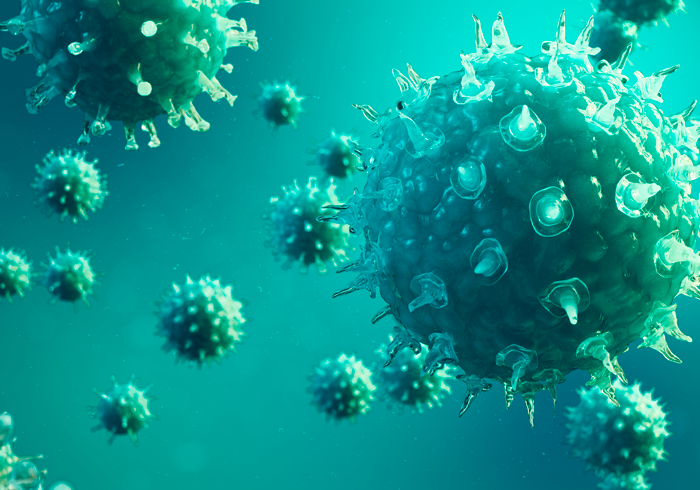
Investigation
Viruses have a secret, altruistic social life
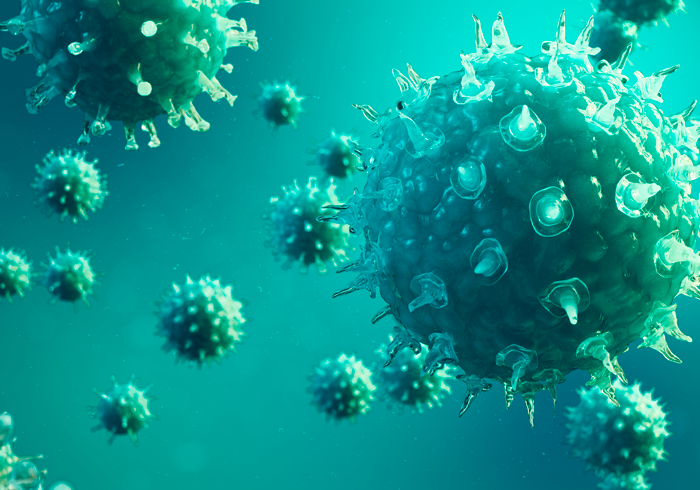
Viruses may seem like the quintessential selfish parasites to their hosts, but researchers are discovering that they can have extensive social interactions with each other, including some behaviors resembling altruism.
Social organisms come in all shapes and sizes, from the obviously social ones like mammals and birds to the more cryptic socializers like bacteria. Evolutionary biologists often confuse altruistic behaviors with each other, because individuals who sacrifice themselves initially appear to be at a serious disadvantage in natural selection. William D. Hamilton, one of the most prominent evolutionary theorists of the 20th century, developed a mathematical theory to explain the evolution of altruism through kin selection, such as why most individual ants, bees, and wasps give up the ability to reproduce and instead pour all their efforts into raising their siblings. Bacteriologists have developed game theory models to explain why bacteria in groups produce metabolites for their neighbors, although some tricksters take advantage of the situation.
But until recently, no one had considered that simple viruses also have social lives that influence their fitness and evolution. “From a theoretical perspective, there is great potential for viruses to interact socially, leading to possibilities for cooperation and conflict,” Stuart West, a biologist at the University of Oxford who studies the evolution of social behaviors, wrote in an email to Quanta. “However, there have been relatively few attempts to address this empirically.”
In a recent study published in Nature Microbiology, Rafael Sanjuán, an evolutionary geneticist at the University of Valencia in Spain, and his colleagues used a combination of theory and experiments to explore viral cooperation and conflict. They found that the spatial structure of a viral infection, the way in which different sets of viruses can be isolated in separate compartments of the infected body, is tremendously important. In a uniformly mixed system, altruistic viruses fall victim to “cheaters” who take advantage of their sacrifices, but if body cavities can isolate and house the altruists, they have a chance to survive.
Consider vesicular stomatitis virus (VSV), a less dangerous member of the same viral family as rabies. Viral infections often stimulate cells in their mammalian hosts to produce interferons, cellular communication that elevate the antiviral defenses of neighboring cells and interfere with viral replication. Wild-type VSV has evolved ways to suppress the innate immune system, but at a slower reproductive cost. Still, that ability allows the population of suppressor viruses to thrive, unless a “trickster” variant appears.
The cheater does not have the ability to suppress its host's defenses; in fact, its presence stimulates the release of interferons. But you still reap the benefit of a reduced immune response due to nearby VSVs suppressing interferon release. Because cheaters do not pay the reproductive cost of interferon suppression, they can outcompete the wild-type virus in the short term. From the point of view of social behavior, as Sanjuán and his colleagues pointed out in their article, the suppression of interferon from wild-type VSV qualifies as an altruistic act because, in effect, the wild type sacrifices itself for the cheater.
Eventually, the host's interferon response overwhelms both types of viruses and kills them. It might seem that natural selection will therefore always eliminate the ability to suppress interferon because its altruism would perversely leave viruses that had it at a disadvantage.
However, Sanjuán's modeling study shows that this is not necessarily the case: the altruistic interferon-suppressing virus can still evolve and thrive if it and the cheater are physically separated. Structures and barriers in the body can create havens where viruses that suppress interferon can survive, safe from the harm that cheaters could cause them.
To model the specific conditions under which innate immune suppression can occur, the researchers used the theoretical framework that Hamilton developed. According to Hamilton's rule, altruism evolves when r × B> C, where B is the benefit to the recipient, r is the relationship of the recipient to the donor, and C is the cost to the donor.
The researchers also used a parameter to indicate that the benefit, B, depends on whether a virus is surrounded by wild-type or cheater neighbors. By applying Hamilton's rule to well-mixed and spatially segregated combinations of the two VSV variants, they could empirically estimate the parameters in Hamilton's equation.
“For innate immune suppression to evolve, a spatial structure is needed,” Sanjuán said. Because both the virus and the host interferon response spread from cell to cell, it is actually quite difficult to prevent the appearance of spatial structures during infection. Limitations on the diffusion rate of viral particles and interferon molecules, as well as physical barriers in body tissues, easily create spatial heterogeneity, allowing the evolution of innate immune suppression.
In animals with complex behaviors and in bacteria with relatively complex communication systems, the outcomes of evolutionary scenarios are influenced by many factors. In the case of viruses, “it is much simpler,” said Sanjuán. "Everything is dictated by the spatial structure. There is no other known process that can affect the outcome of the system. If viruses are mixed, then this altruism cannot evolve, and if they are segregated, then altruism can evolve."
Another aspect of the social evolution of viruses that Sanjuán is investigating is why several viral particles sometimes come together and infect a cell together. The trade-off is that if the viral particles clump together, there are fewer units to infect different cells. So, “in principle, this is expensive because it limits the diffusion capacity,” said Sanjuán. But his team discovered, to their surprise, that the added viruses grow faster and produce more progeny. This result depended on the cell type: in tumor cells that do not have innate immunity, being added was costly. However, in normal cells, which produce an innate immune response, aggregation was beneficial for the viruses because it allows the viruses to overcome the innate immune response, Sanjuán suggested.
Although the aggregation strategy for infection appears beneficial for the virus, it may also lead to the evolution of cheaters. For example, if a virus in the aggregate loses some genes, then it can reproduce faster and, with that advantage, can outcompete the other viruses in the aggregate. These gene-deficient viruses are known as defective interfering particles (DIPs): many of them lack about 90 percent of the viral genome and survive as a small piece of RNA that can replicate very quickly within a host (they generally cannot infect a new virus because they are so incomplete). In cell cultures with a high density of viral infections, DIPs take over and soon represent more than 99 percent of the viral population, Sanjuán said.
The existence of DIPs may address another puzzle: Do viruses adjust their manipulations with each other according to the needs of their life cycle? Raul Andino, a virologist at the University of California, San Francisco, notes that early in its invasion of a host, a virus may want a lot of company because multiple simultaneous infections increase its chances of success. “But then they want to reduce the possibility of a high multiplicity of infection at a later stage, to reduce the possibility of production of these defective particles,” he said. “This is something we don't fully understand, but it is a very interesting problem.”
Source: Viviane Callier - Quantamagazine